Rustom Bhiladvala
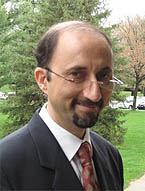
Position
Contact
Credentials
Ph.D (Yale), P.Eng
Area of expertise
Nanoresonator research
Research Areas
- Nanoscale Materials, Mechanics & Transport
- Fluid and Biomedical Sensor Development
- Energy and Sustainable Development
- Nanoscale Synthesis and Fabrication Techniques
Research Overview
In our research effort, we create measurement systems to study fluid-thermal transport, mechanics and material behaviour down to submicron scales. This work provides a basis for the development of biomedical sensors impacting public health and for sensors impacting energy technology. We develop sensors and measurement systems based on nanoscale mechanical devices and microscale thermal sensing devices. This involves the development of materials, nanostructure assembly techniques and measurement techniques. In particular, we focus on methods to enable coverage of large areas by nanostructures at low cost, to remove a hurdle in the practical use of nanotechnology in several application areas.
1. Nanoresonators –Commercial Potential, Experimental Methods, Fields of Study
Vibrating nanowire, fraction of a micrometer in diameter.
One view of the scope of research for nanomechanical resonators (NRS). Commercial potential is shown at top left and experimental methods development in ellipses at left, in yellow. Ellipses at right (green) show a some fields of study used and impacted by our work. Our research, shaped by the awareness of coupling between these areas is aimed at improving practical utilization of results.
Nanoresonators –here, vibrating nanowires, a few hundred nanometers in diameter and a few micrometers in length, are sensitive enough to detect small mass, comparable to that of a few protein molecules or a fragment of DNA. Part of our work is towards translating this extraordinary sensitivity into affordable screening techniques in molecular diagnosis, aimed at the early detection of cancer, genetic abnormalities and serious inflammation. Nanoresonators offer a broad set of opportunities in the development of instruments of great sensitivity, the development of experimental methods in the areas of nanofabrication, transduction (vibration detection and driving) and functionalization (to respond to a specific target). The work uses and extends basic understanding in fields of study such as fluid mechanics, mechanics of materials and non-linear dynamical systems. The challenge in measurements is that the high surface to volume ratio of nanostructures can cause an unusual and strong coupling of physical effects. Sensor platform development must typically reckon with such coupling between transduction schemes, intrinsic stresses from fabrication and nonlinear geometric effects.
2. Development of guard-heated thin-film turbulent wall-shear stress sensors
The sensors described below are being designed for use with wind turbines, for energy savings in transportation, and for better near-wall turbulence models in large-scale computation of flows in nature and in energy conversion system technologies.
Microscale thermal sensors made from thin metal films respond rapidly to changes in temperature or heating/cooling rates. This serves as the basis for the development of a new class of sensors, guard-heated hot-film sensors, to study fluctuations in the shear stress exerted by turbulent flow of a fluid such as air or water past a solid surface (“wall”) - the wall shear stress. This quantity has proved difficult to measure by several techniques, including the use of a conventional single-element hot-film maintained at constant temperature.
We design and fabricate multiple-element thermal sensors (example at left), based on a careful analysis of the errors predicted for conventional single hot-film thermal sensors for wall shear stress. The new sensors are designed to provide missing information and improve the accuracy of turbulent flow models designed to handle large-scale turbulent flow computations at a feasible cost. The sensors are suitable for field use of these devices on structures such as wind turbine blades, providing input for active control systems to reduce failures from extreme load fluctuations in response to sudden changes in wind. Drag reduction and control for aircraft may also benefit from input provided by such sensors.
3. Synthesis of nanostructures and development of assembly techniques
Ordered arrays of nanostructures, with careful design, can contribute, for example to:
- improved efficiency of low-cost organic solar photovoltaics
- improve fuel cell cost, durability and safety by monitoring with in situ distributed sensors within porous media
- allow sensitive detection of a low concentration of multiple disease marker molecules on a single chip, enabling early detection of disease states such as cancers or neurodegeneration
Distributing ordered arrays of nanostructures over large areas involves prohibitive cost if a standard technique such as electron beam lithography is used for patterning. The goal of this effort is to add to a suite of methods to create ordered patterns over large-areas at low-cost, on rigid or flexible surfaces.